
The world of particle physics is a fascinating frontier, constantly pushing the boundaries of human understanding and technology. Among the many captivating elements of this field, one term that often piques curiosity is the “Adam Smasher.” This evocative nickname refers to devices known as particle accelerators, which are central to modern physics research. In this article, we will delve into the Adam Smasher, exploring its history, working principles, notable discoveries, and significance in science and popular culture.
What is an Adam Smasher?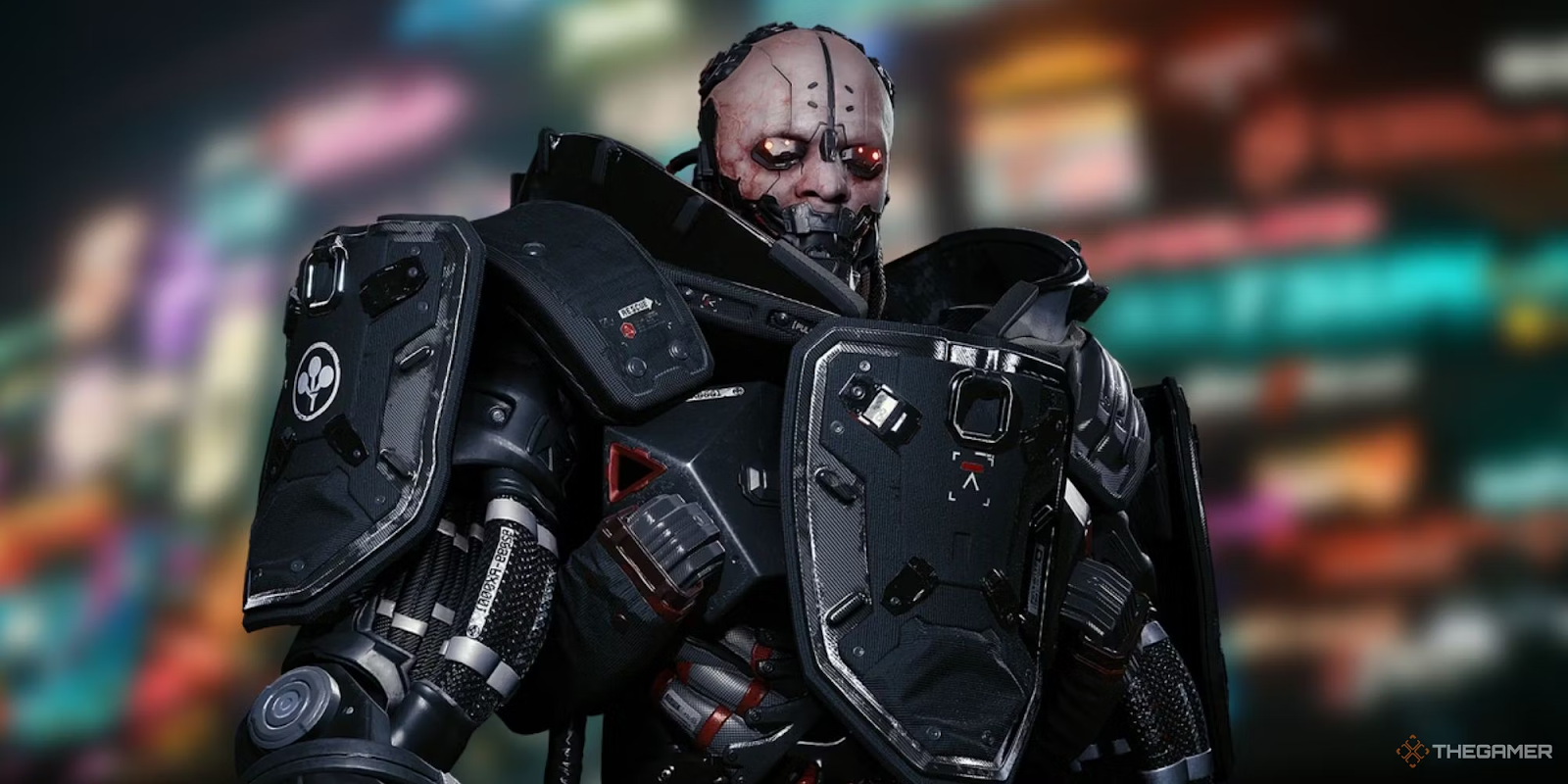
The term “Adam Smasher” is a playful moniker for particle accelerators. These are sophisticated machines designed to accelerate charged particles, such as protons or electrons, to incredibly high speeds and then smash them into each other or into other targets. The resulting collisions release a tremendous amount of energy, producing a variety of subatomic particles. By studying these particles, physicists can gain insights into the fundamental building blocks of the universe and the forces that govern their interactions.
The Evolution of Particle Accelerators
Early Beginnings
The concept of accelerating particles dates back to the early 20th century. The first particle accelerators were linear accelerators (linacs) developed in the 1920s and 1930s. These early devices were relatively simple, using electric fields to accelerate particles along a straight path. However, they laid the groundwork for more advanced machines.
The Rise of Cyclotrons and Synchrotrons
In 1931, physicist Ernest Lawrence invented the cyclotron, a significant leap in accelerator technology. Cyclotrons use a combination of electric fields and magnetic fields to accelerate particles in a spiral path, allowing for higher speeds within a more compact design. Lawrence’s invention earned him the Nobel Prize in Physics in 1939 and opened new avenues for research.
Following the cyclotron, the development of synchrotrons in the 1940s and 1950s marked another milestone. Synchrotrons use a similar principle but can accelerate particles to much higher energies by synchronizing the acceleration process with the increasing particle speed. The ability to reach higher energies made synchrotrons invaluable for exploring high-energy physics.
The Modern Era: Colliders
The most powerful type of particle accelerators today are colliders, where two beams of particles are accelerated and then made to collide head-on. This technique maximizes the energy available for particle interactions. The Large Hadron Collider (LHC) at CERN, operational since 2008, is the world’s largest and most powerful collider. It has been instrumental in numerous groundbreaking discoveries, including the identification of the Higgs boson in 2012.
How Do Particle Accelerators Work?
Basic Principles
At their core, particle accelerators rely on electric and magnetic fields to manipulate charged particles. The electric fields accelerate the particles, while the magnetic fields steer and focus the beams. By carefully controlling these fields, physicists can accelerate particles to speeds approaching the speed of light.
Acceleration Mechanisms
Different types of accelerators use various mechanisms to achieve high speeds:
– **Linear Accelerators (Linacs):** Particles travel in a straight line, passing through a series of accelerating structures.
– **Cyclotrons:** Particles follow a spiral path, gaining energy with each loop.
– **Synchrotrons:** Particles travel in a circular path, with acceleration synchronized to the particle speed.
– **Colliders:** Two beams of particles are accelerated in opposite directions and made to collide.
### Detection and Analysis
Once the particles collide, detectors surrounding the collision point capture the resulting particle debris. These detectors are highly sophisticated, and capable of tracking particle paths, measuring energies, and identifying particle types. The data collected from these collisions is then analyzed using advanced computational techniques to identify new particles and study their properties.
Notable Discoveries and Contributions
### The Higgs Boson
One of the most celebrated achievements of the LHC is the discovery of the Higgs boson. As predicted by the Standard Model of particle physics, the Higgs boson is a fundamental particle associated with the Higgs field, which gives mass to other particles. The confirmation of its existence in 2012 was a monumental milestone, providing critical validation for the Standard Model.
### Quarks and Gluons
Particle accelerators have also been pivotal in uncovering the substructure of protons and neutrons. Experiments conducted at accelerators like SLAC (Stanford Linear Accelerator Center) revealed that protons and neutrons are composed of smaller particles called quarks, held together by particles known as gluons. These findings have been fundamental to our understanding of quantum chromodynamics, the theory describing the strong interaction.
### Antimatter
The study of particle collisions has also contributed to our understanding of antimatter. Antimatter particles are counterparts to regular particles with opposite charges. For instance, the positron is the antimatter counterpart of the electron. Antimatter research has implications for both fundamental physics and practical applications, such as medical imaging techniques like PET scans.
The Impact of Particle Physics on Technology
The advancements in particle physics and the development of particle accelerators have led to numerous technological innovations. Here are some key areas impacted by these developments:
### Medical Applications
Particle accelerators have revolutionized medical imaging and cancer treatment. Techniques, like PET (Positron Emission Tomography) scans, rely on the principles of particle physics to provide detailed images of the human body. Additionally, accelerators are used in proton therapy, a cutting-edge cancer treatment that targets tumours with precision, minimizing damage to surrounding healthy tissue.
Challenges and Considerations
Despite their potential, particle accelerators face significant challenges. The construction and operation of these machines are incredibly expensive and complex. Balancing the cost with the potential scientific benefits is a constant challenge. Additionally, the massive data generated by experiments requires advanced computational resources and sophisticated analysis techniques.
Conclusion
The Adam Smasher, or particle accelerator, remains one of the most powerful tools in the physicist’s arsenal. From unveiling the Higgs boson to exploring the properties of antimatter, these machines have profoundly deepened our understanding of the universe.
Beyond their scientific contributions, particle accelerators have also driven technological advancements and captured the public imagination. As we look to the future, next-generation accelerators promise to unlock even more secrets of the cosmos, continuing the legacy of discovery that has defined particle physics for decades.
FAQs
Q. What is an Adam Smasher?
The term “Adam Smasher” is a colloquial nickname for particle accelerators, which are devices used in physics to accelerate charged particles, such as protons or electrons, to very high speeds. These particles are then collided with each other or with stationary targets to study the resulting particle interactions and the fundamental components of matter.
Q. How does an Adam Smasher work?
Particle accelerators use electric and magnetic fields to accelerate and steer particles. Electric fields provide the force to accelerate the particles, while magnetic fields are used to guide and focus the particle beams. In colliders, two beams of particles are accelerated in opposite directions and made to collide, producing high-energy interactions that can be studied using sophisticated detectors.
Q. What is the purpose of smashing atoms?
Smashing atoms allows scientists to study the fundamental particles and forces that make up the universe. By observing the particles produced in high-energy collisions, researchers can test theoretical models, discover new particles, and gain insights into the properties and behaviour of matter and energy at the smallest scales.
Q. What has the Adam Smasher discovered?
Particle accelerators have led to many significant discoveries, including:
- The Higgs boson: Discovered in 2012 at the Large Hadron Collider (LHC), this particle is crucial for understanding how other particles acquire mass.
- Quarks and gluons: These fundamental particles make up protons and neutrons.
- Antimatter: Studying antimatter has advanced our understanding of fundamental physics and has practical applications in medical imaging.
Q. What is the Large Hadron Collider (LHC)?
The LHC is the world’s largest and most powerful particle collider, located at CERN near Geneva, Switzerland. It consists of a 27-kilometre ring of superconducting magnets and accelerating structures designed to bring protons and heavy ions to nearly the speed of light. The LHC has been instrumental in several key discoveries, including the Higgs boson.
Q. What are some practical applications of particle accelerators?
Beyond fundamental research, particle accelerators have numerous practical applications, including:
- Medical imaging: Techniques such as PET scans rely on principles of particle physics.
- Cancer treatment: Proton therapy uses accelerated protons to precisely target and destroy tumors.
- Materials science: Accelerators help analyze the properties of materials at the atomic level.
- Security: Accelerators are used in cargo scanning systems to detect illicit materials.
Q. How do scientists detect particles after collisions?
After particles collide in an accelerator, detectors surrounding the collision point capture the resulting debris. These detectors are designed to track particle paths, measure their energy, and identify different types of particles. The data collected is then analyzed using advanced computational methods to interpret the results and make new discoveries.
Q. What are the challenges in building and operating an Adam Smasher?
Building and operating particle accelerators is a complex and costly endeavor. Challenges include:
- High construction and operational costs.
- Technical difficulties in maintaining precise control over particle beams.
- Managing the massive amount of data generated by experiments.
- Ensuring the safety and security of the facilities.
Q. What is the future of particle accelerators?
The future of particle accelerators involves developing next-generation machines like the Future Circular Collider (FCC) and the International Linear Collider (ILC). These new accelerators aim to reach even higher energies and greater precision, enabling the exploration of new physics beyond the current capabilities of the LHC.
Q. Why is the Higgs boson important?
The Higgs boson is essential for the Standard Model of particle physics, as it explains how particles acquire mass. Its discovery confirmed the existence of the Higgs field, a fundamental field that interacts with particles to give them mass. Understanding the Higgs boson helps scientists explore the fundamental nature of matter and the universe.
Q.How do particle accelerators contribute to our understanding of the universe?
Particle accelerators allow scientists to probe the fundamental forces and particles that constitute the universe. By recreating the conditions of the early universe and studying high-energy collisions, researchers can test theories of particle physics, explore the properties of matter and energy, and search for new articles and phenomena.
Q. What is CERN?
CERN, the European Organization for Nuclear Research, is a renowned research organization that operates the world’s largest particle physics laboratory. Located near Geneva, Switzerland, CERN is home to the Large Hadron Collider (LHC) and plays a leading role in advancing our understanding of particle physics.
Q. Are there any risks associated with particle accelerators?
Particle accelerators are designed with numerous safety measures to protect both the researchers and the environment. While the high-energy collisions may sound dramatic, the experiments are conducted in controlled environments, and the potential risks are carefully managed. There is no credible scientific basis for concerns that accelerators could create dangerous phenomena like black holes or cause other catastrophic events.
Q. Can particle accelerators be used for energy production?
While particle accelerators are not currently used for energy production, they contribute to research in nuclear fusion, which has the potential to provide a future source of clean and abundant energy. Accelerators help study the conditions necessary for fusion reactions, which could eventually lead to practical fusion energy technologies.
Q. How do particle accelerators impact everyday life?
Particle accelerators impact everyday life in several ways:
- Medical technologies like PET scans and proton therapy improve healthcare.
- Industrial applications, such as materials testing and cargo scanning, enhance safety and quality control.
- Advances in fundamental science and technology from accelerator research contribute to innovations in various fields.
Q. How can the public learn more about particle accelerators?
Many research organizations, including CERN, offer educational programs, tours, and online resources to help the public learn about particle accelerators. Engaging with these resources, visiting science museums, and following scientific news can provide a deeper understanding of the exciting developments in particle physics.
To read more, Click here